ENCYCLOPEDIA OF THE NEW: Spintronics, magnetars and proving relativity
U of M researchers make their mark in physics and astronomy
Kyle Lamothe, Staff
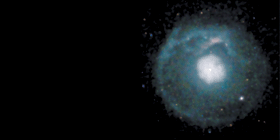
Photo courtesy of NASA/CXC/U.Manitoba/H.Matheson & S.Safi-Harb
When flipping through a textbook, I often wonder about the accomplishments and theories that have contributed to a discipline — the people who made the innovations may have passed on or retired, leaving their work for future generations to study and build upon. I then think of how exciting it must have been to be a student at the time that these discoveries were being made and wonder what it was like to study under professors who were defining the field as we, the students, learned the basics.
But, advancements are constantly being made and built upon in nearly every department, as the world and its people march down the path of development — so what are the profs ranting about around their faculty water coolers, who’s pulling in all the research cash and where is it all going? The “Encyclopedia of New” is a debuting series in the Manitoban that will give you a snapshot into the work of today that will be a highlighted snippet in the textbooks of tomorrow. This week’s department-on-a-pedestal is physics and astronomy.
Dr. Peter Blunden, the head of the department, puts most of the research being done in the Allen Building into three main and broad areas: material science, subatomic physics and astronomy. While there are particles of excitement emitting from every corner of the Allen Building, there’s only enough space for a subatomic look into their work (and a few terrible physics puns).
Nano-everything
In 2000, the federal government set up the Canada Research Chair program, allocating about $900 million to supporting leaders in research. The chair in Nanoscale Physics is at the U of M — Tapash Chakraborty. Chakraborty is at the front of the game in looking at things that are in the size range of a billionth of a metre, where conventional ways of thinking do not apply.
What’s so interesting about studying particles at this tiny scale? Well, not only is it interesting, but it will be the only direction that allows electronics to expand in the coming decades. Transistors, the electronic instruments that run and think for all the gadgets we use, will soon hit a wall that will limit the level of complexity they can reach.
“Transistors are getting smaller and smarter to the point where you cannot see them, because there are millions contained in the chips that are in all electronic devices, ” Chakraborty explained. “We want more and more transistors packed into a smaller space, and at some point there will be problems with heat and other things — and you simply won’t be able to connect them.”
The conventional transistor works by carrying an electrical charge through circuits — by moving tiny particles called electrons in pulses that make up the language of computers. This is how information is stored and how it moves from the buttons on your cell phone through chips to the screen, but there may also be other ways to transfer all those ones and zeroes.
“While electrons move, they also spin — up or down, roughly speaking. Some people are asking whether or not we can transport spin,” he said.
These burgeoning ideas of “spintronics” are still in the theoretical stages, since researchers like Chakraborty and his team are just breaking ground on the hows and whys of the spin of electrons. To answer these questions, research is looking at how the particles react in different conditions, such as in a magnetic field. Eventually, it may be possible to manipulate that spin and transport information based on the position or orientation of the particles when they hit certain points.
Another area in which Dr. Chakraborty works is related to the problem of actually building things that are on such an incredibly small scale. The transistors of today are made with lasers, but we may progress to a point where it will not be possible to construct lasers that are small and precise enough to make the technology we want.
“The important buzz-word in this area right now is ‘self-assembly,’ where you provide an environment so that the components assemble themselves. It is a very ambitious thing. One of the things that people think would be useful in this aspect is a better understanding of DNA,” he said.
Physicists are now delving into the world of complex biological molecules like DNA, studying how and why they behave the way they do. Researchers like Chakraborty are bridging gaps between physics, biology and chemistry, realizing that each discipline is very good at studying certain things, but when brought together, a more complex understanding can be achieved.
A star among astrophysicists
In the relatively new astronomy branch of the department, Dr. Samar Safi-Harb is making a name for herself through her work with the NASA Chandra satellite, which sees the stars using x-raysinstead of just light used by traditional telescopes. Her work with neutron stars has helped answer questions that have had scientists guessing for decades.
When a massive star (much bigger than our sun) reaches the end of its lifetime, it explodes, forming either a neutron star and supernova or a black hole. A neutron star is a smaller version of the original, but it is also an incredibly dense object that is entirely made up of neutrons, the subatomic particles that form with protons and electrons to create atoms. Our knowledge of these neutron stars is relatively limited, as they were only found in the late 1960s and early 1970s.
“Neutrons are very, very dense, so we are talking about a star that is about the size of Winnipeg, but about the mass of the sun — so imagine shrinking the sun into the size of the city,” Safi-Harb explained. “We don’t fully understand the physics of something like that.”
The neutron stars also rotate incredibly quickly, about 30 times a second, and some of them have a ridiculously strong magnetic force.
“I actually believe that these magnetars, as they are now called, are the strongest magnets ever in the universe,” she said. “If one were the distance away from the earth that the moon is, it would pull the change out of your pocket.”
In 2002, Dr. Safi-Harb and her colleagues used x-ray light to measure the magnetic force of a magnetar — something that had never been done outside of theory before.
“So what we are starting to find is that there are different classes of these things. All of the textbooks say that all supernovas should look like the most famous one — the crab nebulae. What we are finding is that this is not the case . . . there is a zoo of neutron stars, and I’m after the zoo and trying to explain the differences between all these different types,” she said.
A lot of Dr. Safi-Harb’s recent work is looking for the clouds of debris around neutron stars that were emitted when the original star exploded. Recently, she made a groundbreaking discovery by finding a cloud around a neutron star that had never been seen before.
Proving Einstein
One major aspect of Einstein’s Theory of Relativity, which is turning 100 years old this year, is the idea that time itself progresses at different rates depending on the speed of the object being examined. U of M’s Dr. Gerald Gwinner and his researchers have proved this in experiments more accurately than anyone else thus far.
Gwinner used a rather simple example to explain this phenomenon . . . but it still doesn’t make it easy for the average person to understand. Imagine two twins, born at almost the same time. One is put on a spaceship that leaves Earth travelling at the speed of light. Since the one on the ship is moving much faster relative to their sibling on earth, time on the craft moves slower. This means that if the ship turned around and travelled back to Earth, the space-kid would be much younger than the one left to age on land.
Noticeable differences such as this only occur when things are moving amazingly fast, such as at the speed of light. Since we can’t actually send a baby out into space on a beam of sunlight, how can this be proven?
“What we do is take a particle and accelerate it to a very high velocity in a particle accelerator. We have tested this so far at seven per cent the speed of light, which requires 7 million volts to accomplish . . . . We then excite the particle with a laser and measure the frequency,” Gwinner explained.
Although this is hard to imagine, Gwinner’s research is important in the science world. His experiments are nearly 10 times more accurate than any other tests ever done on Einstein’s prediction — proving the theory with more certainty.
This research also has practical applications, such as in the Global Positioning System (GPS) in cars, planes and even cell phones today. Since the satellites used in the system are moving very quickly around the earth, their clocks are ticking at different speeds than ours on the ground. If this relative time difference were not accounted for, the system would become about seven kilometres less accurate every day, instead of telling us where we are within metres.
Think your department is doing work that should be added to the Encyclopedia of New? Email tobanfeatures@umanitoba.ca to pitch an idea.